Hypersonic Capabilities
A Journey from Almighty Threat to Intelligible Risk
Lt. Col. Andreas Schmidt, German Air Force
Download the PDF 
According to a lot of open-source publications found in the internet and emphasized by Russian President Vladimir Putin on 4 January 2023, hypersonic capabilities are a force to be reckoned with or even a “game changer” when applied to the stability of the overall international security situation.1 Although hypersonic capabilities are not new, and related technology has been researched since the 1930s, it was almost a century from the first wind tunnel tests of the German “Silbervogel” project to a fielded hypersonic weapon system like Russia’s “Avangard” (claimed operational in December 2019).2 Within NATO, hypersonic capabilities are considered an emerging and disruptive technology, which emphasizes hypersonic capabilities’ evolving nature. This brought the Joint Air Power Competence Centre (JAPCC), NATO’s first and largest center of excellence, into play since it is the mission of a team of multinational experts to provide key decision-makers with effective solutions to air and space power challenges.3 JAPCC sees itself as NATO’s catalyst for improving and transforming joint air and space power, delivering practical solutions through independent thought and analysis.
JAPCC’s subject-matter experts are leading and augmenting NATO and national studies concerning hypersonic capabilities. JAPCC has been supporting the journey from hypersonics as an almost magical “silver bullet” to a threat that can be analyzed with a manageable threat/risk calculus. That does not mean hypersonics do not change the game we have to play into something unwinnable, but it means we can play the game to begin with. The following discussion highlights the path of understanding hypersonics and presents a possible way ahead from defensive and offensive perspectives.
What Are Hypersonic Threats?
By its nomenclature, every threat that moves faster than hypersonic speeds somewhere on its flight path could be considered a hypersonic threat, including most ballistic missiles in their midcourse phase. John D. Anderson, currently the curator for aerodynamics at the National Air and Space Museum, identified five distinguishing characteristics of hypersonic flight: thin shock layers, entropy layers, viscous-inviscid interactions, high-temperature effects and extreme heat transfer, and low-density flows.4 If two or more of these criteria occur at the same time, we consider it hypersonic flight, which generally happens at speeds beyond Mach 5 within the atmosphere. Hence, Mach 5 is not an arbitrary threshold between “supersonic” and “hypersonic,” but it is based on physical phenomena that have demands on the flying object, sufficiently distinguishing it from slower threats.
In 2020, NATO’s Science and Technology Organization Specialist Team 008 (ST008) for Applied Vehicle Technology (AVT) defined a hypersonic vehicle as “flying within the atmosphere for major parts of their non-ballistic trajectory, reaching a velocity of at least five times the speed of sound.”5 Here, hypersonic vehicles were subcategorized into the well-known hypersonic glide vehicle (HGV) and hypersonic cruise missile (HCM). Additionally, the third group of hybrid threats, also called aero ballistic missiles, was defined as representing a weapon between a ballistic missile and an HGV, with characteristics of both. Regardless of whether hypersonic threats are described from a physical or a capability perspective, from a military standpoint, generally, only three aspects matter:
- How survivable is the effector?
- How fast can the effect be delivered?
- Which kind of effect can be delivered?
The better the understanding of hypersonic capabilities, the easier it will be to develop realistic risk calculus. One crucial fact that studying hypersonic threats over the past five years has shown is that we have evolved our understanding and have identified numerous criteria that still require a lot of attention, so the journey needs to continue.
Finally, it should be mentioned that the size of the available hypersonic stockpile is also essential for how and when hypersonic weapons might be employed, but this will not be analyzed in this article.
What Are the Benefits of Having Hypersonic Weapons?
With significant budgetary constraints, no nation will develop and procure new systems when the military benefit does not justify the overall cost. So, fielded hypersonic missiles are either the replacement of an obsolete capability or a dedicated development to create a previously unavailable effect. In the early years of missile development, new systems promised to affect hitherto unreachable targets with little warning times and without endangering the otherwise needed aircrews. Classic examples are ballistic missiles (BM) and cruise missiles (CM).
As the name suggests, the first BM had a simple ballistic flight path, where the main available variables were burnout time and launch angle. Also, gyroscope-based guidance and control systems achieved minor flight path corrections. For example, the accuracy of the German V2 was very limited with a circular error probable (defined as the radius in which 50 percent of the shots land in) of 4.5 km, which does not qualify for any purpose other than terror. In the mid-1980s, the Pershing II medium-range ballistic missile was the first ballistic missile with a truly maneuverable reentry vehicle (MaRV).6 Here, the nonballistic flight path after reentering the atmosphere had two benefits. It allowed the internal guidance sensors more time to acquire the intended target, and it increased survivability against defensive systems.
Consequently, the accuracy and precision could be improved (Pershing II’s circular error probable was 30 m), achieving the intended effect with a smaller payload. Lower circular error probable also allowed the use of ballistic missiles as means of deterrence by denial. Modern ballistic missiles with active guidance sensors promise to hit moving targets to a certain extent. With sufficiently current reconnaissance data, ballistic missiles may be even used against time-sensitive targets.
The first cruise missile built was the German V1 in 1939. At the end of World War II, both German systems (V1 and V2) had a comparable circular error probable, but the V1 was considered the more successful weapon. The speed of effect delivery was relatively unimportant for the pulsejet powered V1. But even with a much lower level of survivability, about 80 percent compared to 100 percent of the V2, it was considered the more effective weapon based on cost and ease of production.7 Modern cruise missiles are faster and have very small circular error probable, which makes them a formidable choice for deliberate planning, deterrence by denial, and time-sensitive targeting.
Hypersonic weapons promise to combine benefits of fast effect delivery and high survivability against modern air and missile defense (AMD) systems. In the past, Putin announced three “invincible” weapon systems that fall into our defined category of hypersonic weapons. Russian videos displayed this superior technology in animated clips and created a lot of hype. Since then, we have learned enough to separate fact from fiction because even emerging threats need to adhere to the laws of physics.
Survivability, Speed, and Range of Hypersonic Threats
Military planners want effectors in their arsenal that have an increased chance of survivability and effect delivery. This reduces the number of systems needed per desired effect compared to more vulnerable legacy systems. To achieve this, hypersonic missiles need to minimize the available battlespace of opposing defensive systems and then minimize the effectiveness of the defensive system in that battlespace. Looking at all currently available AMD systems, hypersonic threats attempt that. HGVs and HCMs have their glide/cruise phase at altitudes between 20 km and 60 km, putting them at the upper fringes of the endo-atmospheric battlespaces of most existing AMD systems. This makes existing interceptors susceptible to the threat system’s maneuverability, especially when having a large speed delta between a hypersonic vehicle and an interceptor. Hypersonic threats have (outside of the boost phase of HGVs) no intersection with exo-atmospheric BMD systems, making them unsuitable for a potential hypersonic AMD system. However, due to the small number of friendly hypersonic missile systems (or adequate representations), existing AMD systems have yet to be tested and verified for defensive capabilities. As of May 2023, Ukraine forces reported to have intercepted multiple Russian Kinzhal missiles with U.S. Patriot AMD systems.8 This might be the first indication of such capabilities against a real hypersonic threat. However, the Kinzhal is an aero-ballistic missile with hypersonic threat features but is not a fully mature hypersonic system. So, the defensive capabilities of Patriot might be akin to the already-known Russian Iskander-M missile system, which has similarities to Kinzhal.
Further analysis will show the implications of this intercept. In general, it is currently more likely that hypersonic threats can be intercepted in their terminal phase, where defensive systems have existing battlespaces against ballistic missiles. Interestingly, HGVs can have two kinds of flight paths. As the name suggests, the glide vehicle can either glide on the upper atmosphere or use a phugoid trajectory, following a wave-like path. The latter complicates the calculation of the terminal phase initiation and heightens the chance of wasted interceptors.
Maneuverability is one factor that increases survivability for all three threat categories of hypersonic weapons. However, every maneuver comes at a cost in either range, speed, or altitude. Maneuver reduces the overall range and potentially increase the risk of interception in the remaining flight path. It is currently unclear whether hypersonic threats can reactively maneuver, which would require onboard sensors and potentially external communications. Preplanned maneuvers require much less energy and, therefore, have a less detrimental effect on the mission. Reactive maneuvers need further analysis of how early an evasive maneuver must be initiated to be effective without hampering the threat’s mission, if possible. The quality of reactive maneuvers will define the requirements for real-time surveillance as well.
As for the effect delivery time, we must identify the speed throughout the flight path. Faster effect delivery times speed up the kill chain and make some formerly unreachable targets viable. HGVs have a depressed ballistic boost phase and an unpowered glide phase (or phugoid trajectory) after reentry, which can extend to intercontinental ranges, depending on the booster and glide capability of the HGV, with speeds up to Mach 20. For sufficient accuracy, the vehicle likely slows below Mach 5 in the terminal phase. The terminal phase of an HGV and HCM will be somewhere between a gradual descent, which would allow for better target acquisition but would allow for more interceptor battlespace, or a relatively abrupt steep descent, which is technically far more complex and, if feasible, very hard to defend.
For HCMs, the vehicle gets boosted to an altitude between 20 km and 40 km when the motor (e.g., Ramjet, Scramjet, or Sodramjet) is powering the cruise and beginning the terminal phases. During the actively propelled phase, the speed is dependent on the actual motor. Ramjet engines could propel an HCM not far beyond Mach 6. Scramjet engines have been tested up to Mach 10 but speeds up to Mach 15 are anticipated. China announced a successful test of a Sodramjet engine with a speed of Mach 16.9 One of the results of the AVT-359 study was that hypersonic motors are susceptible to disruption during strong maneuvers.10 Hence, although the HCM can maneuver, it needs to be within the limits of the engine. This adds to the issue discovered in ST008 that the physical structure of HCMs and HCMs and HGVs may not allow for maneuvers well beyond 10 G, resulting in turning radii of 120 km at Mach 10 and 480 km at Mach 20.11 So, erratic maneuvers cannot be expected. In the terminal phase, HCMs will likely slow down to speeds below Mach 5 due to drag but also because hypersonic motors will probably stop working in a denser atmosphere.
ST008 [NATO’s Science and Technology Organization Specialist Team 008] assessed that hypersonic weapons must slow down below Mach 5 to have sufficient accuracy, leaving an equivalent of only 800 kg TNT, less than a 2,000 lb bomb.
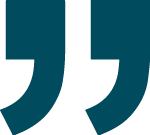
Hybrid threats follow a quasi-ballistic (or aero-ballistic) trajectory, elongated by created lift. Their maximal speed (like those of HGVs) is defined by what the rocket motor can produce at burnout. This is not a new concept. As written above, the Pershing II was the first missile with a MaRV, creating some qualities of hypersonic threats comparable to HGVs, which moved out of focus over the past three decades within the BMD community. However, numerous current systems can be found to employ MaRVs, including Russian Iskander-M, Iranian Soleimani, North Korean KN-23, Chinese Dong Feng 21/26, and U.S. long-range hypersonic weapons. Within this group, the Russian Kinzhal and the Chinese CM-401 are two outliers. Both systems are air-launched BMs, where the actual missile launch point is far more flexible than surface-based systems. Also, the air-launched BMs have an initial launch altitude and speed, positively affecting range and overall speed. Interestingly, and even after several years of international research, the Kinzhal, having similar dimensions and features as the ground-launched Iskander-M and launched from a MiG-31, is supposed to have a range of 2,000 km compared to the Iskander’s range of approximately 500 km. Sometimes in the same articles, the Kinzhal is described to have a range of 3,000 km when launched from SU-22M3 bomber aircraft, which is slower and has a lower max flight ceiling than the MiG-31. A logical explanation is that the Kinzhal’s range includes the operational range of the launch vehicle, which is supported by calculations made in the AVT-359 study, estimating the range increase of an air-launched Iskander variant under perfect conditions will not exceed 150 percent compared to the land-based variant. So far (based on open-source research), aero-ballistic missiles have been the only threats with hypersonic qualities seen in operational use, and their overall numbers seem to be constantly increasing (e.g., RUS Kinzhal, IRN Soleimani, PRK KN23, USA LRHW, etc). This preference for aero-ballistic missiles is likely based on the existing good understanding of BM and the availability of advanced BM technology compared to the new technological fields of HGVs and HCMs. Also, since they are much cheaper to produce than HGVs or HCMs, aero-ballistic missiles can be available in much higher numbers and with a much faster production rate. So, the potential lack of survivability compared to HGVs or HCMs can be compensated by statistics of larger numbers, similar to the V1 and V2 analogy.
Effect Delivery
Understanding how fast and reliable hypersonic weapons can deliver kinetic effects is already quite important, but for operational targeteers, it is even more important to know which kind of effects can be produced. Otherwise, these new expensive tools cannot be smartly used for planning.
The ST008 study calculated that HGVs with strategic range (e.g., Russian Avangard) and HCMs (1,000 km range) could house an effector weighing up to 500 kg.12 These warheads can be either conventional or nuclear. Conventional warheads will often be used for time-sensitive or precision targeting. A 500 kg high-explosive warhead has a very limited surface effect (above ground explosion) with crater sizes below 20 m in diameter and less than 5 m in depth (not considering shrapnel or pressure wave damages).13
Initially, it was thought that hypersonic vehicles would have sufficient kinetic energy at impact to destroy any target. This seems plausible on paper since a 2.5 t HGV impacting at Mach 20 could deliver the same amount of energy as the first nuclear bomb dropped in 1945. However, the HGV will slow down due to drag in the atmosphere. Simulations for the AVT-359 study showed that an HGV could impact somewhere around Mach 7. This would still leave a kinetic energy comparable with approximately 1.5 kt TNT at impact but with a debatable accuracy. ST008 assessed that hypersonic weapons must slow down below Mach 5 to have sufficient accuracy, leaving an equivalent of only 800 kg TNT, less than a 2,000 lb bomb. How deep can it penetrate? Are such effectors limited to surface effects? Following Newton’s approximation for impact depths, the max penetration is about the length of the impactor times the fraction of the impactor density divided by the density of the target material.14 Even specially designed “bunker busters” like the World War II Disney bomb or a more modern GBU-28 have only a penetration depth of about 5 m in reinforced concrete. These bombs, however, have high-density penetration aids to allow this. The GBU-28 casing weighs about 1.8 t, approximately four times more than the assumed HGV/HCM payload of 500 kg. Hence, it can be considered that the penetration capabilities of HGVs and HCMs are significantly less than those of dedicated bunker busters. However, some penetration capabilities will be available, and possibly enhanced through modern tandem charge mechanisms like some cruise missiles (e.g., U.S. Tomahawk Block Vb).15 Discussions with experts in the AVT-359 study pointed out that impacting with hypersonic speeds and fusing at a particular point in time is technically very complex and might run into limitations.
Aero-ballistic missiles’ effects are equal to known BM systems. Historically, they are mainly used for surface effects with relatively low penetration capabilities. However, this knowledge helps targeteers plan these effects since they are well defined in damage and precision.
Deterrence and Protection Considerations
A common understanding of certain concepts like threat, cost, risk, and deterrence is required to support the subsequent arguments. The threat is the combination of capability and the intent to use it. Cost is the negative impact such capability has on our strategic objectives. This can have many metrics like monetary cost, human lives lost, or even the effect on the political/societal will to continue a confrontation. Risk is the chance that a threat will inflict such costs. The goal is to nullify or at least minimize the overall risk for one’s nation or alliance. This can be either done through deterrence or by applying military instruments of power. Deterrence is defined as making a competitor refrain from a particular action based on the predicted cost of this action under specific circumstances. Deterrence occurs both during preconflict and within conflict; restoring deterrence is critical to prevent conflict escalation. Deterrence’s primary purpose is to prevent conflict by pointing out its futility. But deterrence still plays an essential role in case it initially fails by emphasizing the futility of continuing. Deterrence relies on strategic messaging about threatened/predicted outcomes and can help end conflicts earlier. Also, the terms “winning” and “losing” must be defined. Winning happens when one side can maintain sufficient strategic goals to continue a conflict and the competitor cannot. Losing is the opposite, when one side must stop the confrontation due to a catastrophic impact on its strategic goals. However, the transition between the two is gradual, and absolutes are not likely.
In our case, the first step is to negate the possession of hypersonic capabilities themselves by deterring a competitor from developing or procuring them. Nonproliferation architectures like the missile technology control regime already exist for regular missiles and other uncrewed systems.16 It should be feasible to extend such constructs to cover hypersonic capabilities. However, even the missile technology control regime is not a legally binding treaty but an informal political understanding amongst states to limit such proliferations. This gets even more complicated since much modern military technology is dual use with civilian applications. So, nations might be less willing to restrict their global economic influence, even more so for evolving capabilities like hypersonics, which are crucial for any serious national research environment in industry or education to stay competitive. Assuming that the technical availability of hypersonic means over time is hardly preventable, other deterrence goals must be implemented. With a focus on military instruments of power, we must distinguish between means of denial and punishment. Here, deterrence by denial either denies the employment of these weapons prior to launch or negates all their effects afterward with active or passive defense means. An optimized mix of offensive and defensive means must be found to maximize the effectiveness of deterrence by denial. However, since perfect denial is implausible, the remaining risk of such threats must be deterred by means of punishment. Hypersonic weapons can also play an essential role in executing both pillars of deterrence (denial and punishment), which makes friendly possession desirable.17
For the weaker nation in a nonpeer confrontation, hypersonic weapons can be used as means of denial to prevent the opponent from winning in a specific time frame or as a tool for massive punishment as a last resort. The stronger nation in such a competition supports the strategic message of a swift victory, which contributes to deterrence. In times of conflict, hypersonic weapons help to negate adverse capabilities for defense and deterrence and, therefore, end the conflict faster. In a great-power confrontation, hypersonic weapons will likely act operationally or tactically as mission enablers or multipliers by taking out key elements of the adverse military posture. Although this could also be achieved by saturation with less capable means, the psychological effect of Wunderwaffen (wonder weapons) for deterrence and friendly assurance must be taken seriously.18 Therefore, the desire to have hypersonic weapons is there for all competitors.
What can be done to defend against hypersonic weapons? We must look at gaps in the observe, orient, decide, and act loop to enable a defensive structure to intercept hypersonic missiles. Here, we concentrate on observe and act but significant changes in the command-and-control process in between also need to be addressed.
First, the incoming threat needs to be detected and tracked; otherwise, the rest of any kill chain cannot be executed. Consequentially, capability development must start here. Since none of our fielded sensors was developed with this threat in mind, we need to identify which sensors can be adapted and where longer-term gaps might be present. Due to their relatively low flight paths, sensor gaps in the glide/cruise phase are expected, placing the initial emphasis on terminal defense. The physical limitations of hypersonic maneuverability indicate that modern BMD-capable sensor networks should be able to handle hypersonic threats in point defense. However, the impact on the remaining mission set must be researched. Future concepts of space-based infrared satellite networks (e.g., U.S. National Defense Space Architecture) combined with surface-based sensors might close this gap and support a midcourse defense.19 Since modern sensors are software-defined in their capabilities, it is crucial to have the best possible understanding of the threat to optimize the sensor’s search-and-tracking algorithms. This necessitates continuous research on threat development and sufficient information sharing among allies. Only this will allow for adequate defensive requirements, efficient and effective system design, and appropriate defensive employment. Insufficient information sharing could also hamper trust in nondomestic alliance capabilities.
As described above, hypersonic weapons are designed to follow a flight path that minimizes intersection with known defensive battlespaces. All viable intercept points are endo-atmospheric, which excludes any mere exo-atmospheric interceptor like the U.S. SM-3, GBI, or the Israeli Arrow 3. As for endo-atmospheric capabilities, such as THAAD, Patriot, SM-6, or Arrow-2, we must determine the probability of an intercept and how software/hardware changes or an amended firing doctrine could potentially maximize this. The concept of intercepting maneuverable BMs should be familiar to missile defense system designers since MaRV has been a reality for decades. We need the best possible understanding of a threat to optimize the functional requirements for interceptor development. The capability will likely grow from mere point defense to limited area defense. A large area or even territorial defense can only be created by employing a defensive curtain around the area, which may be very cost intensive. However, the industry has already shown creative mitigation options like using air-launched interceptors with fewer capabilities but much more flexible battlespace.
Assuming an interceptor can reach an intercept point, it is vital to identify the most promising form of intercept. A direct hit will likely be 100 percent effective but requires sufficient resilience against maneuvers, making the capability more expensive. Hitting a hypersonic target with very small objects might be sufficient since hypersonic shock effects could allow appropriate damage for ablation to do the rest. Fragmentation warheads are particularly useful as they inflate the impact zone, compensating for insufficient maneuverability in the intercept endgame. This effect is likely factual for a perpendicular impact of a fragment, but effectiveness decreases with shallower impact angles. Differences between glide/cruise and terminal phases must also be identified since the hypersonic vehicle will have slowed down significantly in the latter phase.
Another idea of impacting hypersonic threats is by affecting their guidance and control system. Hypersonic flight depends on stable guidance and control. Even small unforeseen environmental changes could cause compensable and catastrophic effects. So, the idea of sufficiently changing a volume of air by bringing out small particles was conceived and should be further analyzed for practicality and implications on the airspace for friendly use.20 Another way of potentially affecting guidance and control is electronic warfare. In the AVT-359 study, specialists identify which kind of electronic warfare might be practical to achieve such an effect.
Also, lasers or other direct energy weapons are often mentioned as the ultimate interceptor against hypersonic threats. However, hypersonic vehicles are designed to sustain extremely high temperatures to survive hypersonic flight in the atmosphere. The additional energy necessary to have the needed effect on the vehicle structure is exceptionally high. Calculations have shown that only some options for terminal defense over short distances seem currently feasible. This could be a future option for self-defense of high-value assets, especially for those with an unlimited power supply such as nuclear-powered U.S. aircraft carriers. Longer-range intercepts are unlikely in the future due to a lack of sufficient energy projection for having the necessary effect on the target.
As a word of caution, it is imperative not to look at the hypersonic threat in isolation. It is a threat that imposes significant risk, but other threats may be of equal or higher importance when seen in context. Given a likely long-term, high-cost commitment to counter hypersonic threats in a highly budget-constrained environment, it is of utmost importance to make sound decisions on where and how to spend the budget to maximize the stabilizing effect on the security environment or to have an advantage on the battlefield over time. Overly rash decisions for investment to counter hypersonic means might amplify capability gaps in other vital areas, such as counter-unmanned aircraft systems, cyber, or space capabilities. Polemically, one could raise the theory that competitors communicate higher interest in hypersonic weapons to force competing nations into investing resources in own hypersonic or counterhypersonic capabilities, making limited funds unavailable for other critical tasks.
Conclusion and Recommendation
The journey of understanding hypersonic weapons is far from over. Still, over the past few years, through national and NATO studies and research, the fear of a new Wunderwaffe has decreased through furthering our understanding of such enabling technologies. The Mach 20+ “at will” maneuvering weapons have become demystified by analysis of their current and foreseeable capabilities, likely availability, and actual use in conflict. Hypersonic weapons are not the “game changer” competitors want us to believe, but they are dangerous new pieces on the board that need attention. Our initial focus should be on enabling the defense of critical military elements or strategic targets with point defense capabilities. The evolution of current systems should be feasible within the midterm horizon. Also, the required additional sensor network can be used for other military purposes. A credible area defense will remain extremely expensive and resource intensive for the foreseeable future. In parallel, the use of prelaunch offensive means, including the necessary deep sense capabilities and command-and-control networks, must be advanced and deterrence by punishment credibly strengthened. NATO and its nations must ensure that the development of any defensive capability can grow with evolving hypersonic threats. If the procurement process does not provide sufficient flexibility to cover the uncertainty of evolving capabilities (red and blue), it will be behind the power curve in no time.
Lastly, it is critical that these threats not be considered in isolation. Effects can be created in numerous ways within a multidomain environment in an orchestrated fashion. Hence, the perfect hypersonic defense might be nullified by a cheap drone, a sniper, or a fighter aircraft. Military advisors to the procurement process must be honest about the overall risk a threat poses on a timeline to ensure the best mix of capabilities is available to maximize deterrent or fighting postures.
Notes
- Guy Faulconbridge, “Putin Deploys New Zircon Hypersonic Cruise Missiles to Atlantic,” Reuters, 4 January 2023, https://www.reuters.com/world/europe/putin-sends-off-frigate-armed-with-new-hypersonic-cruise-missile-2023-01-04/.
- Tim Robinson, “From Sänger to Avangard—Hypersonic Weapons Come of Age,” Aero Society, 22 October 2019, https://www.aerosociety.com/news/from-saenger-to-avangard-hypersonic-weapons-come-of-age/.
- Joint Air Power Competence Centre, accessed 8 November 2023, https://www.japcc.org/.
- NATO, Assessment of the Status and Challenges Posed by Hypersonic Operational Threats, STO-TR-AVT-ST-008 (Brussels: NATO Science and Technology Organization, August 2020).
- Ibid.
- Encyclopedia Britannica Online, s.v. “strategic missiles,” accessed 9 November 2023, https://www.britannica.com/technology/rocket-and-missile-system/Strategic-missiles#ref521058.
- “Which Was More Successful, the V1 Flying Bomb or the V2 Rocket?,” Quora, https://www.quora.com/Which-was-more-successful-the-V1-flying-bomb-or-the-V2-rocket.
- David Rising, “Ukraine Downs Russian Hypersonic Missile with US Patriot,” Associated Press, 6 May 2023, https://apnews.com/article/russia-ukraine-war-patriot-kinzhal-6b59af8e60853b4d6d16dd8d607768be.
- Stephen Chen, “Chinese Team Test Jet Engine ‘Able to Reach Anywhere on Earth within 2 Hours,’” South China Morning Post (website), 1 December 2020, https://www.scmp.com/news/china/science/article/3111985/chinese-team-test-jet-engine-able-reach-anywhere-earth-within-2.
- NATO, Impact of Hypersonic Operational Threats on Military Operations and Technical High Level Requirements Phase 1, STO-TR-AVT-359 (Brussels: NATO Science and Technology Organization, March 2022).
- NATO, Assessment of the Status and Challenges Posed by Hypersonic Operational Threats.
- Ibid.
- R. D. Ambrosini et al., “Size of Craters Produced by Explosive Charges on or above the Ground Surface,” Shock Waves 12 (2002): 69–78, https://ri.conicet.gov.ar/bitstream/handle/11336/100895/CONICET_Digital_Nro.f7a8b632-1ee4-452b-ba54-69cc1ab0b82d_A.pdf?sequence=2.
- Kyle Hill, “Deep Impact: Newtonian Edition,” Science-Based Life, 15 November 2012, https://sciencebasedlife.wordpress.com/2012/11/15/deep-impact-newtonian-edition/.
- Caleb Larson, “Tomahawk Cruise Missile: A Legend That Can Now Kill Warships,” National Interest (website), 29 March 2021, https://nationalinterest.org/blog/reboot/tomahawk-cruise-missile-legend-can-now-kill-warships-181397.
- “Missile Technology Control Regime (MTCR) Frequently Asked Questions,” Department of State, accessed 9 November 2023, https://www.state.gov/remarks-and-releases-bureau-of-international-security-and-nonproliferation/missile-technology-control-regime-mtcr-frequently-asked-questions/.
- Michal J. Mazarr, “Understanding Deterrence” (Santa Monica, CA: RAND Corporation, 2018), https://www.rand.org/content/dam/rand/pubs/perspectives/PE200/PE295/RAND_PE295.pdf.
- Arnold Blumberg, “Wunderwaffe: The Nazi ‘Wonder Weapons’ of World War II,” WWII History 16, no. 3 (April 2017), https://warfarehistorynetwork.com/article/wunderwaffe-nazi-wonder-weapons-of-world-war-ii/.
- “National Defense Space Architecture (NDSA) Systems, Technologies, and Emerging Capabilities (STEC),” Space Development Agency, 12 January 2023, https://www.sda.mil/national-defense-space-architecture-ndsa-systems-technologies-and-emerging-capabilities-stec/.
- Tom Karako and Masao Dahlgren, Complex Air Defense: Countering the Hypersonic Missile Threat (Washington, DC: Center for Strategic and International Studies, 7 February 2022), https://www.csis.org/analysis/complex-air-defense-countering-hypersonic-missile-threat.
Lt. Col. Andreas Schmidt joined the German Air Force in 1993. After attending officer’s school, he studied computer science at the German Armed Forces University in Munich. Since 1998, he has built an extensive background in ground-based air defense, particularly the Patriot weapon system. He started as a tactical control officer and subsequently held positions as reconnaissance officer, battery executive officer, and battery commander in various Patriot units. Furthermore, he had two nonconsecutive assignments in Fort Bliss, Texas. In between, he had an assignment as the A3C in the former Air Force Division. Currently, he is the integrated air and missile defense/surface-based air and missile defense/ballistic missile defense subject-matter expert at the Joint Air Power Competence Centre.
Back to Top