Preparing for Hot Conflicts
Army Training and Operations in a Warming World
Maj. Erik M. Patton, RG, PhD, U.S. Army
Christopher L. Chapman, PhD
Gabrielle E. W. Giersch, PhD
Download the PDF 
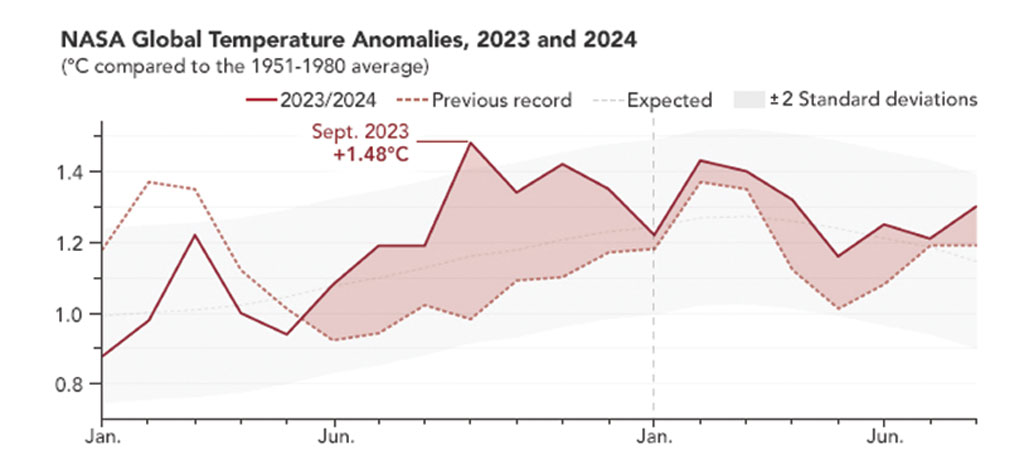
July 2024 was the warmest month ever recorded since widespread reliable recordkeeping began 173 years ago, and the world is now approxi-
mately 2.1oF warmer compared to average temperatures between 1850 and 1900.1 Extreme environmental heat presents a growing challenge to service member health and performance during operations and training. U.S. Secretary of Defense Lloyd Austin has called climate change an “existential threat” to national security—a strong statement from a senior military leader.2 Operationally, an 82oF day may be thought to have no more impact than an 80oF day. However, small changes to average global temperatures create much larger variations in extreme local temperatures, evidenced by worldwide recent “record-shattering” new extreme temperatures.3 In the diverse regions where the Army operates, there is high confidence that hotter local temperatures will continue to occur more frequently and be more extreme than previously recorded.4 Therefore, it is imperative that military leaders understand how the future operational environment will be impacted.
Global climate change, a concept difficult to appreciate in a practical context, will affect the day-to-day training and operations for soldiers in numerous ways. Here, we present an overview of how extreme temperatures impacted U.S. and allied operations over the last twenty years. Additionally, we provide an analysis of the relevance of extreme temperatures to the individual soldier and the operational Army. This analysis predicts that extreme temperatures will vary in intensity locally and heat waves will increase in frequency and intensity until midcentury and likely much longer.
This article is not a treatise on the science behind climate change, nor does it describe all the ways a changing climate will affect national security. These topics are covered at length by others; for military-relevant details, the reader should begin with NATO, Department of Defense (DOD), and individual service documents (e.g., the 2021 “DOD Climate Adaptation Plan,” 2022 United States Army Climate Strategy, or 2023 NATO Climate Change and Security Impact Assessment).5
For simplicity, this article uses the broad term “heat casualty” to refer to soldiers experiencing heat illness during operations or training. Various classifications of heat illnesses, their causes, and preventative measures are described later in the article.
Operational Implications of Extreme Heat
A worst-case heat risk scenario might be a short-notice deployment to an austere urban environment during a humid heat wave. Maximum summer temperatures in many cities already exceed 120°F, and heat-island effects can compound this by adding more than 10°F in some urban locations.6 Acclimatization by progressively increasing work effort in new, hot environments over two weeks is recommended to reduce heat casualties. However, initial response forces likely do not have time for structured acclimatizing to local conditions. Air conditioning in the deployed environment may also be nonexistent, especially if the local power grid is destroyed, leaving just the handful of environmental control units brought with the initial force. Operational considerations likely will require the initial responding force to secure key sites and patrol wearing full kit. Although nighttime temperatures may bring some relief, and operations are likely to be conducted at night whenever possible, this presents a trade-off with rest cycles as sleeping during the daytime heat can impact sleep and recovery.7 In such a scenario, heat quickly becomes a critical operational factor.
The Army has guidelines for operating in the heat, but these work-rest cycles designed to maximize performance while also mitigating against heat casualty risk cannot be followed if the military situation does not allow it.8 This complication was observed by a British military doctor in southern Iraq who described that although temperatures well exceeded thresholds to trigger work-rest cycle guidelines during most of the day, military necessity required continuous operations. Even low-risk duties performed in extreme temperatures became high risk as pragmatism and an emphasis on treating heat casualties effectively overtook work-rest cycle recommendations as the way to balance operational necessity with heat casualty risk.9
Operational impacts of such a scenario extend to mission planning. Patrolling with gear or other “heavy work” can inhibit the body’s ability to shed heat fast enough to prevent core temperature from rising when wet bulb globe temperature (WBGT) index exceeds about 78°F.10 At this temperature, the Army’s heat stress control manual recommends continuous heavy exertional efforts such as patrolling be limited to two hours or less. Such limitations might not be practical for an initial responding force as exceeding 78°F on the WBGT index is common even in current non-heat wave summer conditions. For example, since 2009, Fort Moore in Georgia experiences about half of all hours in July and August at or above this threshold.11 Guidance suggests additional constraints on military operations at higher temperatures, recommending time spent on continuous patrol be less than one hour when the WBGT exceeds 88°F. Above 90°F, “training may require extreme modifications or temporary suspension.”12 The challenge is that some activities, such as security, cannot be suspended. Thus, operations in extreme temperatures struggle to balance the risk of heat casualties with mission requirements.
In nonpermissive environments, heat casualty prevention is deprioritized compared to immediate military necessity, which may increase the risk for larger numbers of heat casualties. A NATO report found that in August 2004, 10 percent of the force engaged in Najaf, Iraq, became “incapacitated from heat stress and [were] evacuated.”13 During that battle, temperatures reached 130°F, and thirst was at times a higher priority than enemy fire. Tanks crews rotated by section to recover from the heat and were administered intravenous fluids to help prevent heat casualties, with some crew members hanging two or three IV bags inside their tanks while fighting.14 Similar situations were observed during dismounted engagements in Afghanistan, where maneuver was slowed by heat and necessary treatment of heat casualties.15
Vehicle crew members, who compared with dismounted soldiers operate with relatively less physical exertion, can also become heat casualties. A 2007 Canadian Army study found expected temperatures inside the Leopard 2C tanks operating around Kandahar to be higher than 145°F, conditions that would render the crew “operationally impaired within 1-2 hours.”16 Microclimate cooling using air-cooled vests was recommended to improve operational effectiveness, but whole-vehicle cooling was considered impractical. In armored personnel carriers, dismounts may not have access to cooling vests and are likely to remain challenged by high temperatures during transport.
In permissive or semipermissive environments, heat illness may contribute to a substantial portion of overall casualties. During 2003 in southern Iraq, 161 of 766 British soldiers admitted to the field hospital for heat illness were evacuated back to the United Kingdom, with this “large number of personnel being evacuated [causing] concern amongst the operational staff.”17 In Afghanistan, heat illness was the second most common reason for internal medicine admissions at the Role 3 British hospital in Camp Bastion.18 Based on British experiences, during summer expeditionary operations in austere environments “approximately 50 per 1,000 troops deployed” can be expected to be heat casualties in the first ten to fourteen days of operations.19 This rate would result in the temporary loss of more than a company equivalent of soldiers during the first two weeks of a brigade-size response. Most heat casualties can expect to return to duty but require a period of recuperation; although nearly 80 percent were discharged back to their unit, the average hospital stay for a British heat casualty during the first twelve months of conflict in Iraq was 2.9 days followed by an additional limited-duty profile period.20 Table 1 provides a list of select studies and reports that describe heat casualties in military operations.
How Extreme Heat Negatively Affects Soldier Health and Performance
“Heat illness” is the general term in medical literature for negative heat-related health outcomes. Exertional heat illness exists on a spectrum with myriad signs and symptoms (see the figure).21 Heat exhaustion is considered the least severe form of exertional heat illness, and soldiers typically return to duty after a few days of recovery. Heat injury is characterized by hyperthermia, that is, an elevated body core temperature, with evidence of end-organ damage (e.g., kidneys, liver, muscle, gastrointestinal) and may require two weeks or more for recovery depending on the extent of the injury. Heat stroke is a severe and potentially fatal form of exertional heat illness that presents with hyperthermia (usually > 104°F rectal temperature) and central nervous system disturbances and may cause multiorgan damage or failure. After a heat stroke, Army Regulation 40-501, Standards of Medical Fitness, proscribes a ten-week return-to-duty process that may be lengthened (or shortened) based on medical provider judgment.22
Every soldier has the fundamental limitation of being human, subject to a physiological ceiling for adapting to extreme environments that cannot be overcome by fitness or motivation. Soldiers must prevent their body core temperature from rising excessively or risk death by heat stroke. This can become challenging when performing tasks such as patrolling, conducting physical training, and other daily work. Metabolic rates increase in proportion to exercise intensity during physical activity, with some metabolic energy converted into mechanical energy but most released as heat produced by working skeletal muscles during exercise.23 Working at the same rate, a soldier with better movement economy, not aerobic fitness, will produce less metabolic heat and have smaller heat storage compared to a less efficient counterpart.24
Increased heat storage during physical work challenges soldier health and performance. During physical work in hot environments, cardiac output (i.e., the amount of blood pumped by the heart per minute) increases to support the metabolic demand of working muscles.25 However, to support thermoregulation in these conditions, blood flow is also redirected away from visceral organs to support thermoregulation and heat loss mechanisms.26 The risk of non-heat stroke end-organ damage is increased with hyperthermia and dehydration, believed to primarily occur due to this reduced blood flow to these vital organs.27 During exertional heat stroke, elevated body temperature is accompanied by central nervous system dysfunction (e.g., delirium and combativeness), often with end-organ damage similar to that observed with heat injury.
Unfortunately for soldiers, the laws of physics limit heat loss rates when high temperatures occur with high humidity. Sweating is the most effective form of heat dissipation, with heat drawn from the skin as sweat evaporates. The ability to lose heat via sweating can be impaired by high humidity, low air velocity, or clothing, especially impermeable clothing like chemical, biological, radiological, nuclear, and explosive (CBRNE) protective equipment. This creates conditions under which unrestrained increases in body core temperature occur that cannot be compensated by physiological processes like sweating, and the risk of exertional heat illness is significantly increased.
In temperatures measured by the wet-bulb thermometer—which, like a sweating human, is cooled by evaporation—work becomes difficult above a wet bulb temperature of 88°F and potentially fatal at temperatures not much higher (see table 2).28 Historically, a wet bulb temperature of 95°F was proposed as the theoretical thermal human survival limit. At this threshold, heat loss from the body effectively stops but heat storage from the environment and metabolism continues, leading to heat illness.29 Recent studies have challenged this 95°F limit by finding that much lower wet bulb temperatures (~81°F) create conditions under which heat loss can’t keep pace with heat generated from low intensity activities like walking.30 To account for the cooling power of sweat and the influence of humidity, along with the need to consider solar radiation (which adds an additional heat burden) and wind speed (which can promote cooling), the military uses the WBGT index to classify dangerously high heat. Spikes in soldier heat casualties have been associated with periods of raised humidity even as ambient temperatures remained relatively constant, and humid Army installations in the South and Southeast routinely have greater rates of heat illness than hotter but drier locations in the desert Southwest.31
As body core temperature rises, especially with intense physical activity during high WBGT, heat stroke risk is increased. During training, heat stroke is almost exclusively of the exertional variety. Pacing strategies, such as slowing down or utilizing work-rest cycles, can effectively mitigate excessive heat strain and help to prevent exertional heat stroke.32 However, these strategies are not always an option. No soldier wants to quit on their teammates or themselves, leading to the paradox that high motivation is a heat stroke risk factor in soldiers.33 Timed ruck marches and runs are some of the events with the highest incidence of heat casualties.34 Fear of falling out, getting cut from a course for missing a standard, or motivation to lead from the front can cause a soldier to override their natural protective instinct to slow down. Fort Moore, Georgia, has the highest reported incidence of heat stroke across all U.S. military installations—fifty-six cases in 2023—due to a combination of environmental conditions (e.g., temperature and humidity) and motivational factors (training effort during infantry, armor, airborne and Ranger courses). Of note, the higher incidence of exertional heat stroke at Fort Moore is partly from the enhanced recognition and accurate reporting of cases due to ongoing training and support provided by the Army Heat Center within Martin Army Community Hospital.
During operations, nonexertional heat illness contributes to a large proportion of heat casualties, especially during the first couple weeks deployed in a hot environment. As the name suggests, these heat illnesses aren’t associated with heavy work, and acclimatization and environmental factors are more relevant than working effort. The British Army, analyzing heat casualties during operations in Oman, Afghanistan, and Iraq, found nonexertional heat illnesses accounted for most (up to 86 percent) cases observed in soldiers recently arriving to the area of operations.35
An Ever-Hotter Battlefield
Climate change impacts in one part of the world can lead to ripple effects around the world, affecting U.S. security and economic interests. Climate change is expected to contribute to political and social instability and, in some cases, conflict, affecting a wide range of U.S. national security interests.36 Given the Army’s worldwide presence, soldiers will be required to operate in parts of the world where the impacts of climate change are most severe. Average global temperatures have increased by over 2°F, and this increase elicits greater temperature extremes.37 Heat waves will significantly affect future Army operations as they increase in frequency, duration, and intensity.38 Presently, heat waves across the United States are twice as common and the heat wave season is thirty days longer than it was in the 1980s.39 Even under relatively optimistic projections, heat waves like those that killed tens of thousands in Europe in 2003 and Russia in 2010 are likely to occur in the United States in the coming decades and more often in other parts of the world.40 Worldwide, heat waves with an intensity that occurred on average once every ten years will be 5.6 times as likely and nearly +5°F more intense (above already hot historical maximum temperatures) when the world experiences an additional 2°F of warming.41 Put plainly, the frequency of exceptionally hot days is rising much faster than gradual warming of global temperature would suggest.
Heat waves directly impact operations. Black flag days, when the Army’s top heat safety threshold is exceeded, result in training modification or rescheduling.42 In summer 2023, Joint Base San Antonio, Texas, experienced seventy-four days of 100+ degree weather, and over half of all hours in the month of July experienced temperatures exceeding the “heat risk” threshold set by the National Weather Service.43 Combat medic trainees removed vests and helmets as temperatures climbed and afternoon missions were cancelled.44 At the Joint Readiness Training Center in Louisiana, the most common summer training injuries that 1st Battalion, 5th Aviation Regiment’s medevac unit responds to are heat related.45 Over the last five years, 84 percent of the installations tracked by the Defense Centers for Public Health had more exceptionally hot heat risk days than the fifteen-year average.46
Abroad, heat waves increasingly affect areas critical to U.S. security interests, including where soldiers are currently or have recently deployed. Last year, Basra exceeded 123oF, and Baghdad reached 120oF. A 2024 RAND corporation study points to the multiple recent heat waves in Basra and Baghdad as a contributing factor to insecurity and rioting. The study finds parts of Syria and Jordan may experience “extreme danger” heat classification, defined as periods when heat stroke is highly likely, for fifteen or more days per year by midcentury. In fact, “nearly all countries in the [CENTCOM] AOR are increasingly exposed to extreme heat,” and Iran may experience an incredible thirty additional days at the extreme danger threshold.47 Globally, at least twenty-three countries have already recorded maximum temperatures above 122oF.48
Ever increasing temperatures will be the norm for the foreseeable future. Heat waves are predicted to significantly increase over most of Africa, South America, and Southeast Asia in the near term, with heat wave events rare or unprecedented today becoming normal in some future scenarios.49 Estimates using current warming trends project an additional 2.0–4.5°F increase in global average temperature this century above the approximately 2°F already experienced.50 At a global 3.6°F increase (approximately half of which has already occurred), the frequency of extreme heat waves is expected to double over most of the world and affect nearly 40 percent of world population once every five years.51
Adapting to Ensure Future Readiness
Military operations won’t stop on account of extreme heat, yet human physiology limits how far a soldier can push before becoming a heat casualty. Future conditions will increase challenges for soldiers operating during heat waves more intense than those experienced during home station training. The remainder of this article proposes adaptation measures to ensure the Army can fight and win wars in the future, hotter operational environment.
To an extent, adaptations already occurring have proved successful in reducing the most severe heat illness. In 2022, U.S. Army Training and Doctrine Command (TRADOC) recorded the lowest number of heat strokes in the last ten years (ninety total), and 2023 had the third lowest (111), even as those years had the seventh and third highest rate of heat illness overall.52 This reduction to the most severe form of heat illness during training is likely due to a combination of safety measures, policies, command emphasis, and widespread adoption of techniques like arm immersion cooling.53 Perhaps the best example of heat stroke mitigation is the 47 percent decrease in this condition at Fort Moore, Georgia, since the Army Heat Center stood up there in 2019.54 Similar efforts at other training installations may yield similar results for other training programs.
While heat illness is an acknowledged and mitigated risk in TRADOC, training programs in TRADOC are highly structured and facilitate relatively easy adoption of risk mitigation practices. Less attention has been given to how the operational Army can adapt to rising heat. Rapid, initial responses during real-world operations have less flexibility to incorporate safety precautions implemented in training. Even the Army’s medical manual on heat illness prevention acknowledges “work cycles should be customized for the environment, task, and military situation when possible [emphasis added].”55
The most basic, and one of the most effective, risk mitigation method is to address individual risk factors associated with heat illness. For soldiers, modifiable risk factors include physical fitness and body mass. Higher body mass index increases risk for exertional heat stroke, while more fit individuals have lower risk of heat illness.56 The physiological reasons are many, but in general terms, excess body fat impairs heat loss and increases heat storage, while increased cardiovascular fitness is associated with increased sweat rate and blood flow that can help shed excess body heat.57 Three other individual factors are worth mentioning: hydration, medication, md motivation. Unsurprisingly, a dehydrated soldier is more susceptible to heat illness and end-organ damage, although hydration alone does not prevent it.58 Medication is more complicated and may not be controllable if a soldier requires certain prescriptions, but it is important to note that some classes of common medications can reduce the body’s ability to shed heat.59 Lastly, high internal motivation to excel in soldiers has recently been demonstrated to be an important risk factor for exertional heat stroke, especially during high risk events such as timed runs or rucking.60
For units, the most effective available adaptation is acclimatizing all members to the heat. Ten to fourteen days of progressively longer work in the heat promotes physiologically protective adaptations within the body. The rates of these cardiovascular and thermoregulatory adaptations vary, but maximum benefits are achieved after about the second week, and fit soldiers acclimatize fastest.61 In some military situations, acclimatization can be challenging or even impossible; for example, it would be impractical for paratroopers stationed at Joint Base Elmendorf-Richardson, Alaska, to properly heat acclimatize prior to a jump onto an airfield in tropical Guam (as was conducted in June 2020). Acclimatization efforts, typically a structured program of physical training/work in the heat, must be balanced against predeployment tasks, time available, and the risk of overtraining. NATO recommendations call for two hours per day of cardiovascular endurance exercise in the heat to achieve acclimatization.62 Despite the challenges, acclimatization programs should be conducted whenever possible before deploying to hot environments. If unable to acclimatize predeployment, the first two weeks in the hot environment should be structured to provide gradual acclimatization whenever practical.63
Operationally, units can adapt to extreme heat by operating primarily at night. During extreme heat waves, operating at night may be the only practical way to reduce an unacceptably high risk of heat casualties during missions. Fortunately, this adaptation aligns well with elements of the soldier lethality concept defined in the 2019 Army Modernization Strategy, specifically the development and fielding of next-generation night vision.64 The Army has always claimed to own the night, and in future operating conditions where extreme heat degrades daytime operations, fighting at night may be the norm. Predeployment training should reflect this, with nighttime training emphasized, at least during the summer. Further, heat casualty identification and response should be a battle drill rehearsed prior to deployment by every soldier with an emphasis on a “cool first, transport second” approach based on the need to rapidly reduce body core temperature in a heat casualty.
Logistically, adaptations for operating in hot environments fall into two categories: unit equipment and medical equipment. For unit equipment, air conditioning in vehicles must be operational. Every troop-carrying vehicle should be equipped with coolers for ice sheets. Ice making equipment should be obtained and prioritized for early deployment; it is notable the 2023 National Defense Authorization Act lists containerized ice making systems as a directed priority item for National Guard and Army Reserve procurement alongside aircraft, drones, and communication equipment.65 Ice machines may not rank highly as a capability considered critical for operations, but they can save lives by providing the means to restock ice sheets and medical sites for fast cooling of heat casualties, particularly when ice cannot be sourced locally. Ice packs should be stocked with the assumption that use for cooling (especially in the absence of ice production) will consume greater than normal quantities of this mundane medical supply in hot environments, and a platoon can more easily distribute many ice packs among soldiers instead of carrying larger ice blanket coolers during dismounted operations.
For the medical community, the British example of an enhanced Role 1 in Kuwait is illuminating.66 This enhanced Role 1 included a heat illness unit to support troops deploying to Iraq in summer 2003 that was equipped with nonstandard items like spray bottles and electric fans to cool heat casualties. The heat illness unit handled three hundred heat-related casualties in one month of operation and provides a template for modifying U.S. Role 1 facilities deploying to hot environments. During operations in Oman, British medical personnel discovered the environmental control unit (ECU) organic to the medical tent was unable to adequately cool the interior, resulting in temperatures regularly exceeding 86oF; local air conditioners were purchased to supplement the inadequate ECU.67 Similarly, British Land Rover battlefield ambulances used in Kuwait were unable to cool the patient compartment below 95oF even when operating at full capacity and were supplemented with a nontactical air-conditioned vehicle. While it is unclear if U.S. ECUs and field ambulances have more robust air conditioning systems, effective air conditioning must be a consideration for medical teams deploying in hot environments.
During severe future heat waves, current equipment may prove inadequate. Humid heat approaching the limits of human tolerance may already be occurring for short durations in a few hot coastal locations; some future projections anticipate this condition will regularly occur in parts of the world soon after 2050.68 In such conditions, more extreme adaptations may need to be developed. One potential is personal biometric sensors. Wearable devices to predict imminent heat stroke have already been developed by the U.S. Army Research Institute of Environmental Medicine and employed in select training groups.69 Advanced development of such sensors could integrate directly into a soldier’s equipment, allowing real-time assessment of core temperature and predicting imminent heat casualties. Understanding just how hard a unit can push before taking heat casualties may extend the operational range of soldiers. Coupled with personal cooling devices, future soldiers may be able to operate in conditions that an adversary can’t, gaining a distinct tactical advantage. Although variations of personal cooling technology exist, such equipment is not currently issued, and some systems (such as small personal air conditioners) are practical only with CBRNE or explosive ordnance disposal suits. Further refinement may lead to practical individual cooling systems to augment the body’s natural cooling ability. Small, unmanned ground vehicles can assist with heat management by hauling heavy supplies during patrols, reducing effort (and therefore heat generated) by soldiers who would otherwise carry the equipment. This technology is also not advanced enough for field deployment but may soon be. If operational environments reach conditions where even moderate physical effort results in a dangerous body core temperature increase, a more robotic future may be the only practical way to conduct daytime operations. It remains to be seen if such autonomous systems can be developed and fielded before soldiers must operate in such an extreme environment.
Conclusion
Climate change will continue to adversely affect temperatures, creating future operating conditions more extreme that those the Army has experience operating in. Seemingly small increasing global temperatures will result in heat waves occurring more frequently, at greater intensity, and in parts of the world that have not experienced extreme temperatures. Although extreme heat has always been a challenge, soldiers will be expected to operate in conditions that are hotter, and hotter for longer, than historically experienced during previous campaigns or training.
Extreme heat degrades both individual soldier and unit effectiveness. Physiology, physics, and experience all show that hot—especially hot and humid—weather increases the risk for heat casualties, including potentially fatal heat stroke. For individual soldiers, both exertional and nonexertional heat illness present risk. Exertional heat illness accounts for many of the heat injuries experienced during training when working effort generates large metabolic heat loads, particularly during runs and ruck marches. Nonexertional heat illness is more common during the first two weeks of deployment in hot environments before a soldier has acclimatized to new conditions. For units deployed or training in hot environments, operational effectiveness is degraded when heat casualties occur. The ability of tactical units to maneuver and fight is reduced during high temperatures for both mounted and dismounted soldiers. Commanders and staff may need to balance the risk of heat casualties against operational risk, especially during an initial response to hot environments when there is no time for structured acclimatization. Recent global operations highlight the risk of heat illness are not trivial, and adaptations are occurring in some training environments, but adaptations to mitigate heat risk during deployment are less developed and should be aggressively pursued.
The opinions or assertions contained herein are the views of the authors and are not to be construed as official or as reflecting the views of the U.S. Army, the Department of Defense, or the U.S. government.
Notes
- Sally Younger, “NASA Finds Summer 2024 Hottest to Date,” NASA, 11 September 2024, https://www.nasa.gov/earth/nasa-finds-summer-2024-hottest-to-date/.
- Lloyd Austin, “Statement by Secretary of Defense Lloyd J. Austin III on the Department of Defense Climate Adaptation Plan,” U.S. Department of Defense, 7 October 2021, https://www.defense.gov/News/Releases/Release/Article/2803761/statement-by-secretary-of-defense-lloyd-j-austin-iii-on-the-department-of-defen/.
- E. M. Fischer, S. Sippel, and R. Knutti, Increasing Probability of Record-Shattering Climate Extremes,” Nature Climate Change 11, no. 8 (2021): 689–95, https://doi.org/10.1038/s41558-021-01092-9.
- Working Group I, Intergovernmental Panel on Climate Change, “Summary for Policymakers,” in Climate Change 2021: The Physical Science Basis, ed. V. Masson-Delmotte et al. (New York: Cambridge University Press, 2021), 3–32, https://doi.org/10.1017/9781009157896.001.
- Austin, “Department of Defense Climate Adaptation Plan”; Office of the Assistant Secretary of the Army for Installations, Energy and Environment, United States Army Climate Strategy (Washington, DC: Department of the Army, February 2022); NATO Secretary General, NATO Climate Change and Security Impact Assessment (Brussels: NATO, 2023).
- Ahmed Rizwan, Leung Dennis, and Chunho Liu, “A Review on the Generation, Determination and Mitigation of Urban Heat Island,” Journal of Environmental Sciences 20, no. 1 (2008): 120–28; Nadja Popovich and Christopher Flavelle, “Summer in the City Is Hot, but Some Neighborhoods Suffer More,” New York Times, 9 August 2019, https://www.nytimes.com/interactive/2019/08/09/climate/city-heat-islands.html.
- Nick Obradovich et al., “Nighttime Temperature and Human Sleep Loss in a Changing climate,” Science Advances 3, no. 5 (26 May 2017): e1601555, https://doi.org/doi:10.1126/sciadv.1601555.
- Technical Bulletin, Medical (TB MED) 507, Heat Stress Control and Heat Casualty Management (Washington, DC: U.S. Government Publishing Office [GPO], 12 April 2022), 18–19, https://armypubs.army.mil/epubs/DR_pubs/DR_a/ARN35159-TB_MED_507-000-WEB-1.pdf.
- Unpublished analysis of data provided by the Army Heat Center, Fort Moore, Georgia, March 2024.
- TB MED 507, Heat Stress Control and Heat Casualty Management, 60.
- Unpublished analysis of data, March 2024.
- TB MED 507, Heat Stress Control and Heat Casualty Management, 17.
- Technical Report AC/323(HFM-187)TP/496, Management of Heat and Cold Stress Guidance to NATO Medical Personnel (Brussels: NATO, December 2013), 55, https://www.sto.nato.int/publications/STO%20Technical%20Reports/RTO-TR-HFM-187/$$TR-HFM-187-ALL.pdf.
- Francis Kozlowski, “The Battle of An-Najaf” (monograph, Washington, DC: History Division, U.S. Marine Corps, 1 July 2004), 41, https://apps.dtic.mil/sti/citations/AD1122724.
- John McGrath, “Operation STRONG EAGLE: Combat Action in the Ghakhi Valley,” in Vanguard of Valor: Small Unit Actions in Afghanistan, ed. Donald Wright (Fort Leavenworth, KS: Combat Studies Institute Press, 2012), 110, https://www.armyupress.army.mil/Portals/7/combat-studies-institute/csi-books/VanguardOfValor.pdf.
- Ira Jacobs et al., Heat Stress Mitigation for Leopard 2C Tank Crew (Toronto: Defence Research & Development Canada, May 2007), i, https://apps.dtic.mil/sti/citations/ADA472970.
- M. Bricknell, “Prevention of Heat Illness in Iraq,” Proceedings of the RTO Human Factors and Medicine (HFM) Panel Special Meeting (Boston: NATO HFM, 2004).
- Andrew Cox et al., “A 2-Year Review of the General Internal Medicine Admissions to the British Role 3 Hospital in Camp Bastion, Afghanistan,” BMJ Military Health 162, no. 1 (2016): 56–62, https://doi.org/10.1136/jramc-2014-000385.
- J. P. Bolton, P. H. Gilbert, and C. Tamayo, “Heat Illness on Operation Telic in Summer 2003: The Experience of the Heat Illness Treatment Unit in Northern Kuwait,” BMJ Military Health 152, no 3 (2006):148–55, https://doi.org/10.1136/jramc-152-03-07.
- C. Grainge and M. Heber, “The Role of the Physician in Modern Military Operations: 12 Months Experience in Southern Iraq,” BMJ Military Health 151, no. 2 (2005): 101–4, https://doi.org/10.1136/jramc-151-02-08.
- William Roberts et al., “ACSM Expert Consensus Statement on Exertional Heat Illness: Recognition, Management, and Return to Activity,” Current Sports Medicine Reports 20, no. 9 (2021): 470–84, https://doi.org/10.1249/jsr.0000000000000878.
- Army Regulation 40-501, Standards of Medical Fitness (Washington, DC: U.S. GPO, June 2019), 31, https://armypubs.army.mil/epubs/DR_pubs/DR_a/ARN37720-AR_40-501-002-WEB-4.pdf.
- Matthew Cramer et al., “Human Temperature Regulation Under Heat Stress in Health, Disease, and Injury,” Physiological Reviews 102, no. 4 (2022): 1907–89, https://doi.org/10.1152/physrev.00047.2021.
- Jovana Smoljanić et al., “Running Economy, Not Aerobic Fitness, Independently Alters Thermoregulatory Responses during Treadmill Running,” Journal of Applied Physiology 117, no. 12 (2014): 1451–59, https://doi.org/10.1152/japplphysiol.00665.2014.
- Cramer et al., “Human Temperature Regulation.”
- Christopher Chapman et al., “Kidney Physiology and Pathophysiology during Heat Stress and the Modification by Exercise, Dehydration, Heat Acclimation and Aging,” Temperature 8, no. 2, (2020): 108–59, https://doi.org/10.1080/23328940.2020.1826841.
- Christopher Chapman et al., “Both Hyperthermia and Dehydration during Physical Work in the Heat Contribute to the Risk of Acute Kidney Injury,” Journal of Applied Physiology 128, no. 4 (2020): 715–28, https://doi.org/10.1152/japplphysiol.00787.2019; Zachary Schlader et al., “The Potential for Renal Injury Elicited by Physical Work in the Heat,” Nutrients 11, no. 9 (2019), https://doi.org/10.3390/nu11092087.
- Jonathan Buzan and Matthew Huber, “Moist Heat Stress on a Hotter Earth,” Annual Review of Earth and Planetary Sciences 48, no. 1 (2020): 623–55, https://doi.org/10.1146/annurev-earth-053018-060100; table from “Exertional Heat Illness Prevention for Service Members” (Falls Church, VA: Defense Health Agency, 2023), 2, https://ph.health.mil/PHC%20Resource%20Library/cphe-heat-ehip-guide.pdf.
- Steven Sherwood and Matthew Huber, “An Adaptability Limit to Climate Change Due to Heat Stress,” Proceedings of the National Academy of Sciences 107, no. 21 (2010): 9552–55, https://doi.org/doi:10.1073/pnas.0913352107.
- Daniel Vecellio et al., “Greatly Enhanced Risk to Humans as a Consequence of Empirically Determined Lower Moist Heat Stress Tolerance,” Proceedings of the National Academy of Sciences 120, no. 42 (2023): e2305427120, https://doi.org/doi:10.1073/pnas.2305427120; Daniel Vecellio et al., “Critical Environmental Limits for Human Thermoregulation in the Context of a Changing Climate,” Exercise Sport and Movement 1, no. 2 (2003), https://doi.org/10.1249/esm.0000000000000008.
- Bricknell, “Prevention of Heat Illness in Iraq”; U.S. Army Training and Doctrine Command (TRADOC), TRADOC Heat Illness Report: 2023 Annual Report (Fort Eustis, VA: TRADOC, 2024).
- TB MED 507, Heat Stress Control and Heat Casualty Management; Thomas Deshayes et al., “Work-Rest Regimens for Work in Hot Environments: A Scoping Review,” American Journal of Industrial Medicine 67, no. 4 (2024): 304–20, https://doi.org/10.1002/ajim.23569.
- Faith Alele et al., “Epidemiology of Exertional Heat Illness in the Military: A Systematic Review of Observational Studies,” International Journal of Environmental Research and Public Health 17, no. 19 (2020), https://doi.org/10.3390/ijerph17197037.
- Julien Périard, David DeGroot, and Ollie Jay, “Exertional Heat Stroke in Sport and the Military: Epidemiology and Mitigation,” Experimental Physiology 107, no. 10 (2022): 1111–21, https://doi.org/10.1113/ep090686.
- Cox et al., “A 2-Year Review of the General Internal Medicine Admissions”; Samuel White and M. B. Smith, “Effects of Heat: UK Exercise Saif Sareea 3 and Interpreting Military Climatic Guidance,” BMJ Military Health 166, no. 6 (2020): 401–5, https://doi.org/10.1136/bmjmilitary-2019-001356.
- M. E. Hellmuth et al., “Climate Effects on US International Interests,” in Fifth National Climate Assessment, ed. A. R. Crimmins et al. (Washington, DC: U.S. Global Change Research Program, 2023), 17-8–17-12, https://doi.org/10.7930/NCA5.2023.CH17.
- Working Group I, “Summary for Policymakers,” 3−32.
- International Federation of Red Cross and Red Crescent Societies (IFRC) and UN Office for the Coordination of Humanitarian Affairs (OCHA), Extreme Heat: Preparing for the Heatwaves of the Future (Geneva: IFRC/OCHA, October 2022), https://www.ifrc.org/sites/default/files/2022-10/Extreme-Heat-Report-IFRC-OCHA-2022.pdf.
- Kate Marvel et al., “Climate Trends,” chap. 2 in Crimmins et al., Fifth National Climate Assessment.
- Simone Russo et al., “Magnitude of Extreme Heat Waves in Present Climate and Their Projection in a Warming World,” Journal of Geophysical Research Atmospheres 119, no. 22 (2014): 12500–12, https://doi.org/10.1002/2014JD022098.
- Sophie Boehm and Clea Schumer, “10 Big Findings from the 2023 IPCC [International Panel on Climate Change] Report on Climate Change,” World Resources Institute, 20 March 2023, https://www.wri.org/insights/2023-ipcc-ar6-synthesis-report-climate-change-findings.
- Erik Patton et al., “Wet Bulb Globe Temperature from Climate Model Outputs: A Method for Projecting Hourly Site-Specific Values and Trends,” International Journal of Biometeorology 68, no. 12 (December 2024): 2663–76, https://doi.org/10.1007/s00484-024-02776-5.
- Douglas Holl, “Climate Change Impacts Heat Risk,” Health.mil, 22 March 2024, https://www.health.mil/News/Dvids-Articles/2024/03/22/news466878.
- Sig Christenson, “It’s Been So Hot at Camp Bullis, the Army Has Cut Trainees Some Slack,” San Antonio Express-News, 18 September 2023, https://www.expressnews.com/news/article/army-training-intense-heat-camp-bullis-18283369.php.
- Jean Graves, “BJACH, Cajun Dustoff, Support Readiness, Rotational Units at JRTC,” Defense Visual Information Distribution Service, 14 June 2023, https://www.dvidshub.net/news/447151/bjach-cajun-dustoff-support-readiness-rotational-units-jrtc.
- Holl, “Climate Change Impacts Heat Risk.”
- Michelle Miro et al., A Hotter and Drier Future Ahead: An Assessment of Climate Change in U.S. Central Command (Santa Monica, CA: RAND Corporation, 29 November 2023), 31, https://doi.org/10.7249/RRA2338-1.
- Mohammed Haddad, “Mapping the Hottest Temperatures Around the World,” Al Jazeera, 1 July 2021, https://www.aljazeera.com/news/2021/7/1/interactive-mapping-hottest-temperatures-around-world.
- Russo et al., “Magnitude of Extreme Heat Waves.”
- IPCC, Climate Change 2023: Synthesis Report. Contribution of Working Groups I, II and III to the Sixth Assessment Report of the Intergovernmental Panel on Climate Change (Geneva: IPCC, 2023), https://www.ipcc.ch/report/sixth-assessment-report-cycle/.
- Alessandro Dosio, “Extreme Heat Waves Under 1.5°C and 2°C Global Warming,” Environmental Research Letters 13, no. 5 (25 April 2018): 1–10, https://iopscience.iop.org/article/10.1088/1748-9326/aab827/pdf.
- TRADOC, TRADOC Heat Illness Report.
- David DeGroot, Robert Kenefick, and Michael Sawka, “Impact of Arm Immersion Cooling During Ranger Training on Exertional Heat Illness and Treatment Costs,” Military Medicine 180, no. 11 (2015): 1178–83, https://doi.org/10.7205/milmed-d-14-00727.
- Ashish Vazirani, Report to the Committees on Armed Services of the Senate and the House of Representatives: Heat Illness Report (Washington, DC: U.S. Department of Defense, November 2023), 14, https://www.health.mil/Reference-Center/Reports/2023/11/28/Heat-Illness-Report.
- TB MED 507, Heat Stress Control and Heat Casualty Management, 17.
- Gabrielle Giersch et al., “Body Mass Index, but Not Sex, Influences Exertional Heat Stroke Risk in Young Healthy Men and Women,” American Journal of Physiology: Regulatory Integrative and Comparative Physiology 324, no. 1 (12 December 2022): R15–R19, https://doi.org/10.1152/ajpregu.00168.2022; Sheryl Bedno et al., “Exertional Heat Illness Among Overweight U.S. Army Recruits in Basic Training,” Aviation, Space, and Environmental Medicine 81, no. 2 (1 February 2010): 107–11, https://doi.org/10.3357/asem.2623.2010.
- Cramer et al., “Human Temperature Regulation.”
- Christopher Chapman et al., “Acute Kidney Injury Biomarkers and Hydration Assessments Following Prolonged Mild Hypohydration in Healthy Young Adults,” American Journal of Physiology: Renal Physiology 325, no. 2 (July 2023): f199–f213, https://doi.org/10.1152/ajprenal.00086.2023.
- Edward Ashworth, James Cotter, and Andrew Kilding, “Methods for Improving Thermal Tolerance in Military Personnel Prior to Deployment,” Military Medical Research 7, no. 1 (2020): 58, https://doi.org/10.1186/s40779-020-00287-z.
- Kirsten Lalli et al., “The Role of Motivation to Excel in the Etiology of Exertional Heat Stroke,” Journal of Special Operations Medicine 24, no. 2 (June 2024): 28–33, https://doi.org/10.55460/4tiv-hqlo.
- NATO, Management of Heat and Cold Stress.
- Ibid.
- Ashworth, Cotter, and Kilding, “Methods for Improving Thermal Tolerance.”
- Army Futures Command, Army Modernization Strategy (Washington, DC: U.S. GPO, 2019), 6, https://www.army.mil/e2/downloads/rv7/2019_army_modernization_strategy_final.pdf.
- House Appropriations Committee, Department of Defense Appropriations Bill, 2023, H.R. Rep. No. 117-388 (2022), https://www.congress.gov/congressional-report/117th-congress/house-report/388/1.
- Bolton, Gilbert, and Tamayo, “Heat Illness on Operation Telic in Summer 2003.”
- White and Smith, “Effects of Heat: UK Exercise Saif Sareea 3.”
- Russo et al., “Magnitude of Extreme Heat Waves.”
- Kyla Driver et al., Physiological Monitoring of the Marine Corps Recruiting Depot - Parris Island, SC Crucible Events (Fort Detrick, MD: U.S. Army Medical Research and Development Command, 27 March 2023), https://apps.dtic.mil/sti/citations/trecms/AD1199011.
Maj. Erik Patton, RG, PhD, U.S. Army, is an engineer with the U.S. Army Security Assistance Training Management Organization at Fort Liberty, North Carolina, and a recent graduate of the Advanced Strategic Planning and Policy Program, where he received his PhD at Duke University researching implications of climate change on military training. He holds a BS from the U.S. Military Academy, MS degrees from Missouri University of Science and Technology and Kansas State University, and a master’s in operational studies from the U.S. Army Command and General Staff College. He has served with the 1st Infantry Division, 1st Armored Division, 25th Infantry Division, and the U.S. Army Corps of Engineers.
Christopher Chapman, PhD, is an Oak Ridge Institute for Science and Education postdoctoral research fellow at the U.S. Army Research Institute of Environmental Medicine. He received his PhD in exercise and nutrition sciences from the State University of New York at Buffalo and completed a postdoctoral fellowship in human physiology at the University of Oregon funded by the National Institutes of Health. His research has largely focused on the pathophysiological responses to physical work during extreme heat.
Gabrielle Giersch, PhD, is a research physiologist in the Thermal and Mountain Medicine Division at the U.S. Army Research Institute of Environmental Medicine (USARIEM). Before becoming a research physiologist, she was a postdoctoral fellow at USARIEM. She holds a BS from Roanoke College, an MS from James Madison University, and a PhD from the University of Connecticut. Her research at USARIEM investigates thermoregulation, exertional heat stroke risk factors, return to duty, and female warfighter physiology in extreme environments.
Back to Top